Leaf temperatures, traits, and rates
A grand challenge for humanity is predicting how climate change will alter the functioning of the biosphere and the services it provides. Current predictive models suffer from two main limitations. First, most models assume that plant physiology is driven by ambient macroclimate, but this is almost never accurate. Instead, plant traits operate via energy budgets to decouple macroclimate from the microclimates at which physiological processes occur. For example, plant leaves and forest canopies buffer variation in ambient temperature, which promotes maintenance of plant temperatures near metabolic optima and provides thermal refugia in variable climates. Second, most models are based on tissue, organ, or organismal physiology, so their predictions do not easily map to higher levels of organization (such as communities and ecosystems) that experience spatial variation in microclimate and physiological traits. For example, while climate has a well-established influence on rates of leaf-level carbon assimilation, it has a much weaker influence on rates of ecosystem-level carbon assimilation.
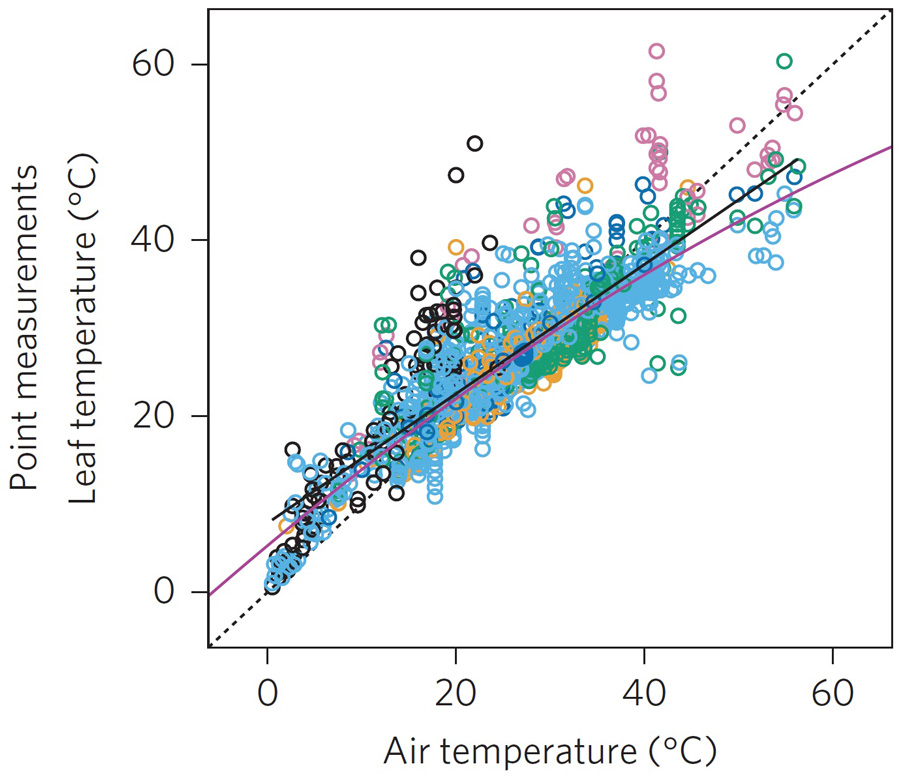
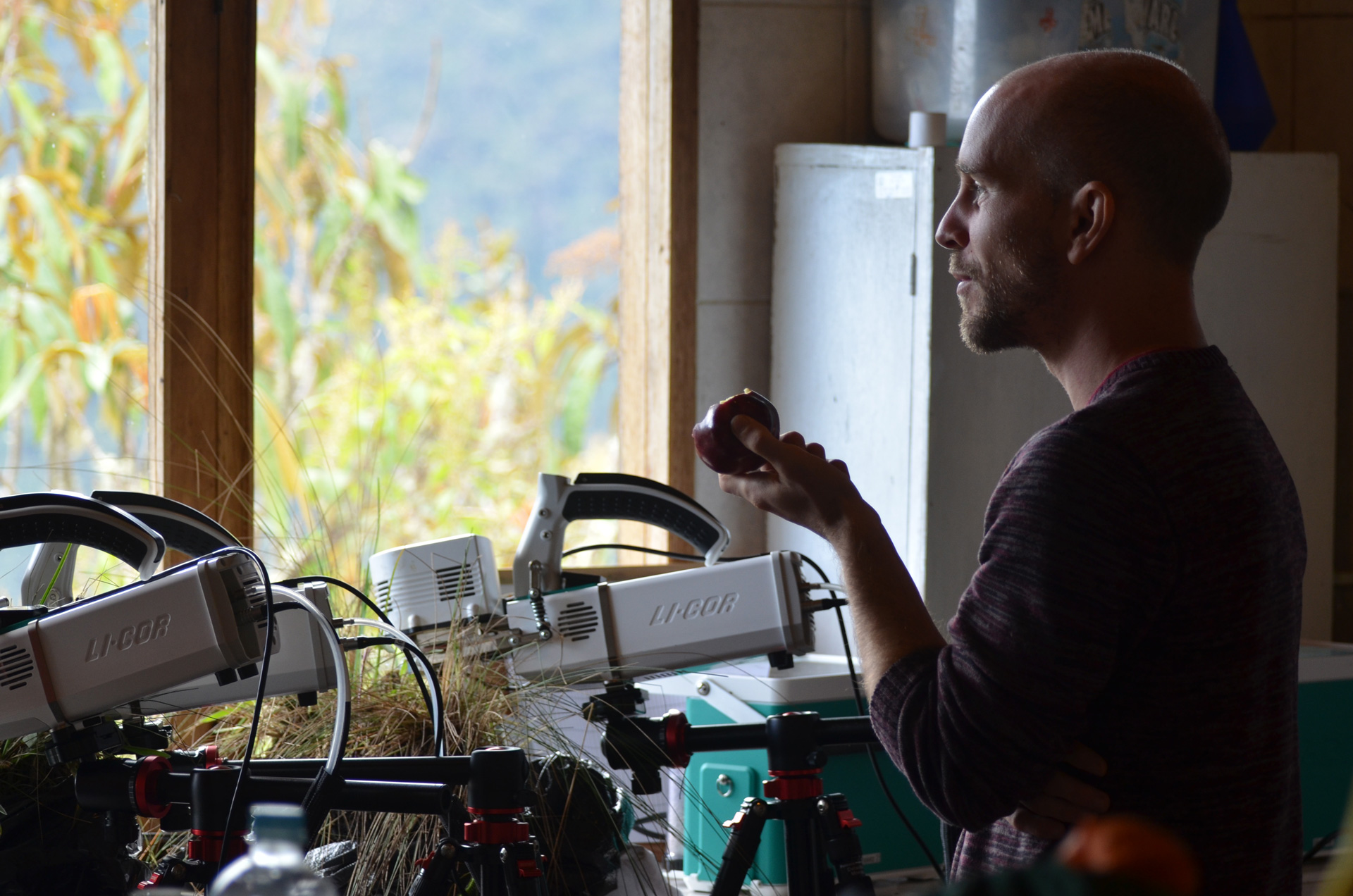
Predicting how spatially and biologically complex natural communities will respond to climate change requires a mechanistic mathematical framework for scaling plant-environment interactions across levels of organization, linking small scale climate and physiology with large scale ecosystem dynamics. We are synthetizing approaches from meteorology and scaling to develop novel integrative theory for predicting plant microclimates from macroclimate and forest structure. Microclimatic variation then drives variation in plant physiological rates, which “scale up” to control higher level processes such as primary productivity and evapotranspiration.
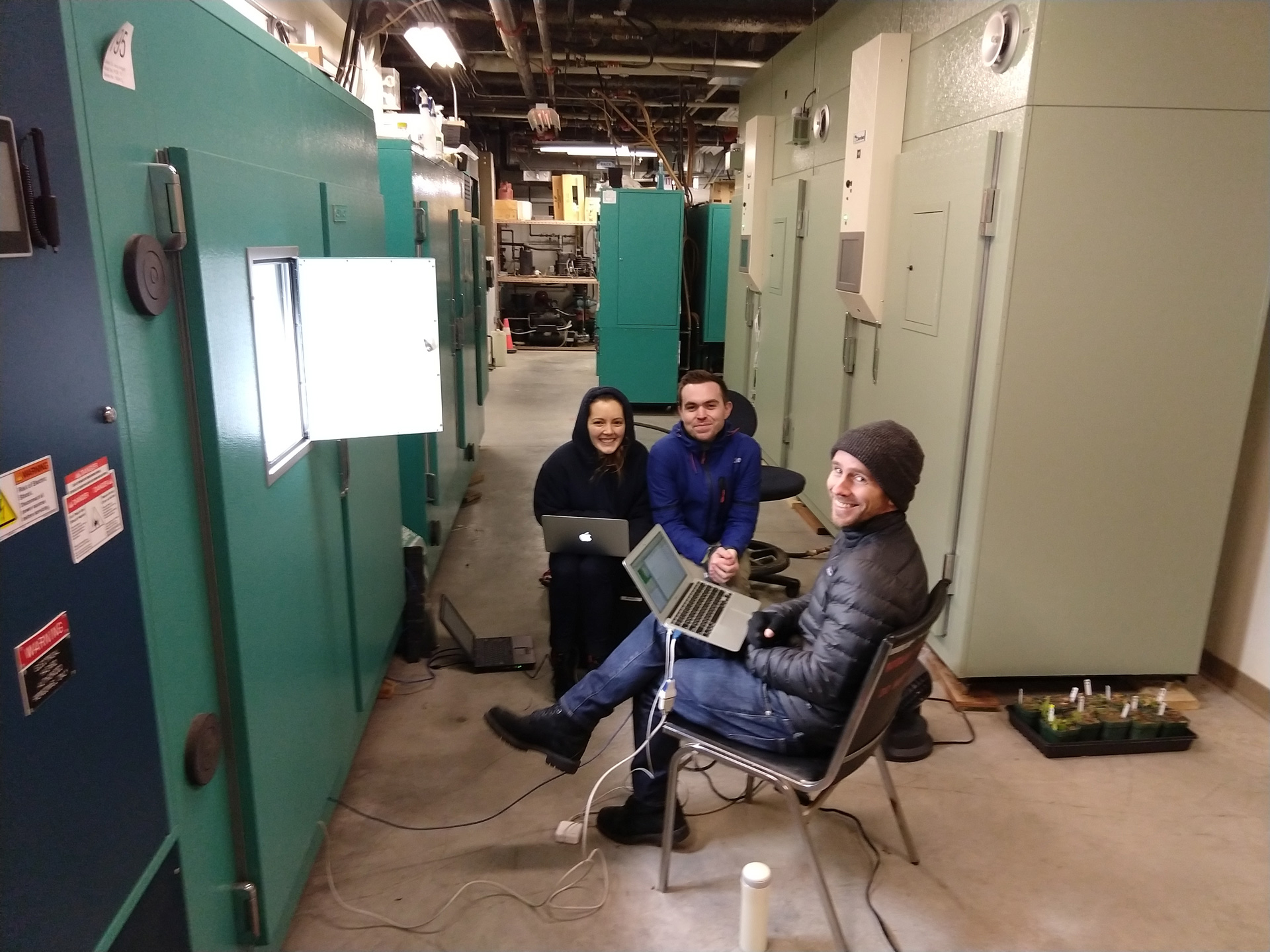
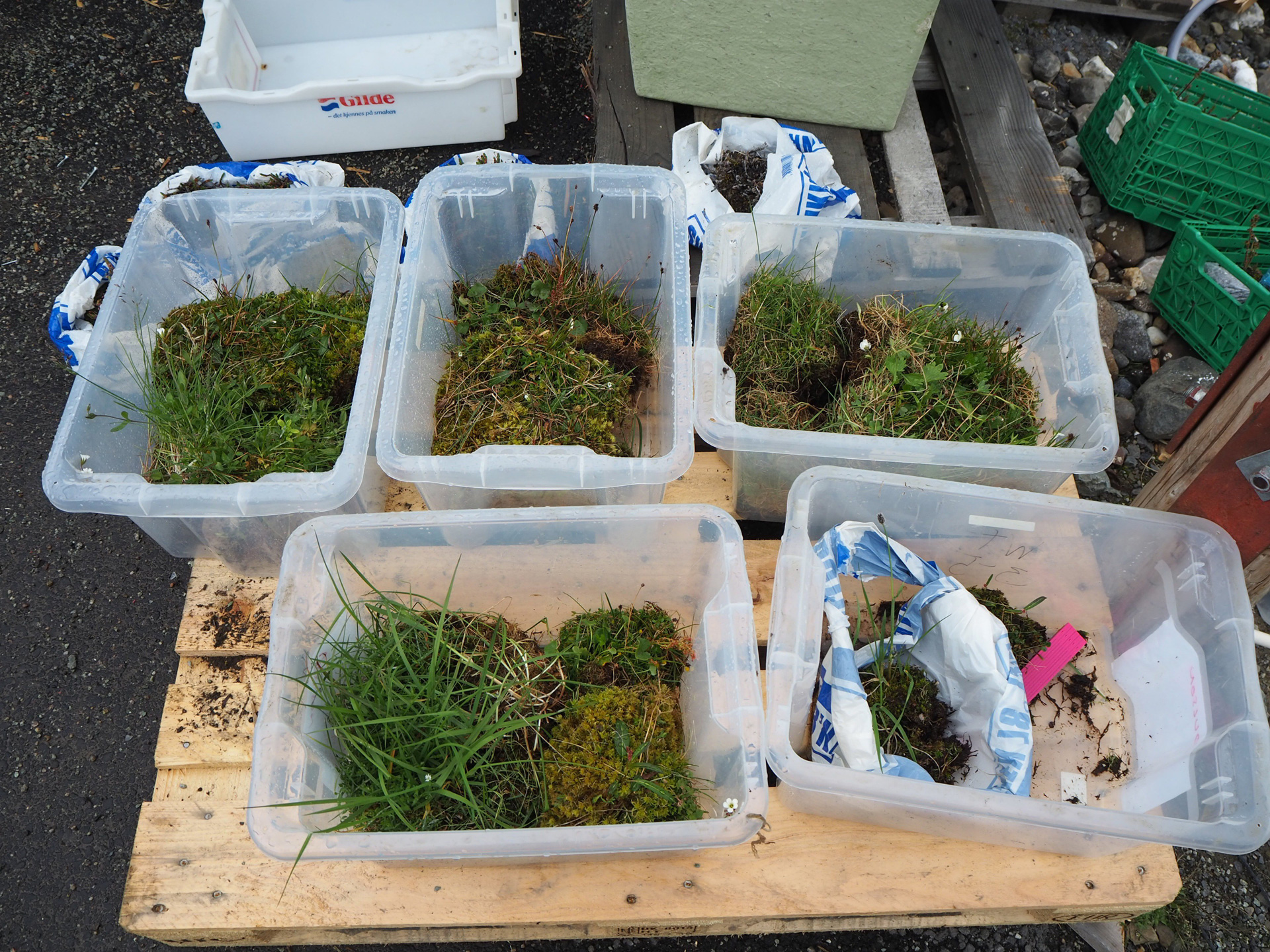
Key resources
Garen JC, Michaletz ST. In press. Acclimation unifies the scaling of carbon assimilation across climate gradients and levels of organization. Ecology Letters. Full text, Data, Code
Bison NN, Michaletz ST. 2024. Variation in leaf carbon economics, energy balance, and heat tolerance traits highlights differing timescales of adaptation and acclimation. New Phytologist 242:1919-1931. Full text, Supporting Information, Data and code
Michaletz ST, Garen JC. 2024. Hotter is not (always) better: Embracing unimodal scaling of biological rates with temperature. Ecology Letters 27:e14381. Full text, Supporting Information, Data and code
Garen JC, Michaletz ST. 2024. Fast Assimilation-Temperature Response: A FAsTeR method for measuring the temperature dependence of leaf-level photosynthesis. New Phytologist 241:1361-1372. Full text, Data and code
Garen JC, Aparecido LMT, Blonder BW, Cavaleri MA, Slot M, Michaletz ST. 2023. Canopy-top measurements do not accurately quantify canopy-scale leaf thermoregulation. Proceedings of the National Academy of Sciences of the USA 120:e2301914120. Full text
Garen JC, Branch HA, Borrego I, Blonder B, Stinziano J, Michaletz ST. 2022. Gas exchange analyzers exhibit large measurement error driven by internal thermal gradients. New Phytologist 236:369-384. Full text, Supporting Information, Data and code
Blonder B, Escobar S, Kapás RE, Michaletz ST. 2020. Low predictability of energy balance traits and leaf temperature metrics in desert, montane and alpine plant communities. Functional Ecology 34:1882-1897. Full text, Supporting Information
Blonder B, Michaletz ST. 2018. A model for leaf temperature decoupling from air temperature. Agricultural and Forest Meteorology 262:354-360. Full text, File S1, File S2, Mathematica code, R code, Data
Michaletz ST. 2018. Evaluating the kinetic basis of plant growth from organs to ecosystems. New Phytologist 219:37-44. Full text, Supporting Information, R code
Forest MacroSystems network
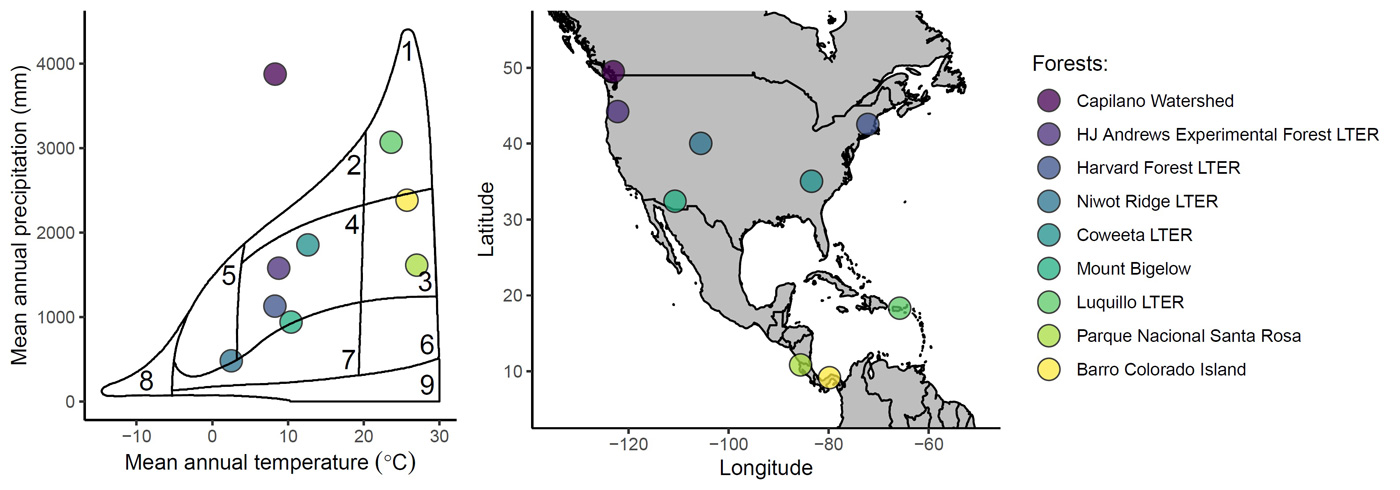
The Forest MacroSystems (FMS) network is a set of long-term forest monitoring plots that provide data for analyzing and understanding drivers of global variation in forest diversity, demographics, and dynamics. It was established in 2011 in collaboration with the Enquist Macroecology Lab and several others. The FMS network currently comprises nine sites arrayed across a latitudinal climate gradient, from tropical seasonal forest in Panama (9° N) to temperate rainforest in British Columbia (50° N). The network is expanding; please contact Sean Michaletz if you are interested in installing a site!
We are currently working on several projects based on FMS network data. One project synthesizes radiative transfer theory and metabolic scaling theory to quantitatively predict energy non-equivalence in forest communities, and test this using FMS data. Another project is examining the influences of plant size, plant functional traits, and climate on global variation in forest mortality rates.
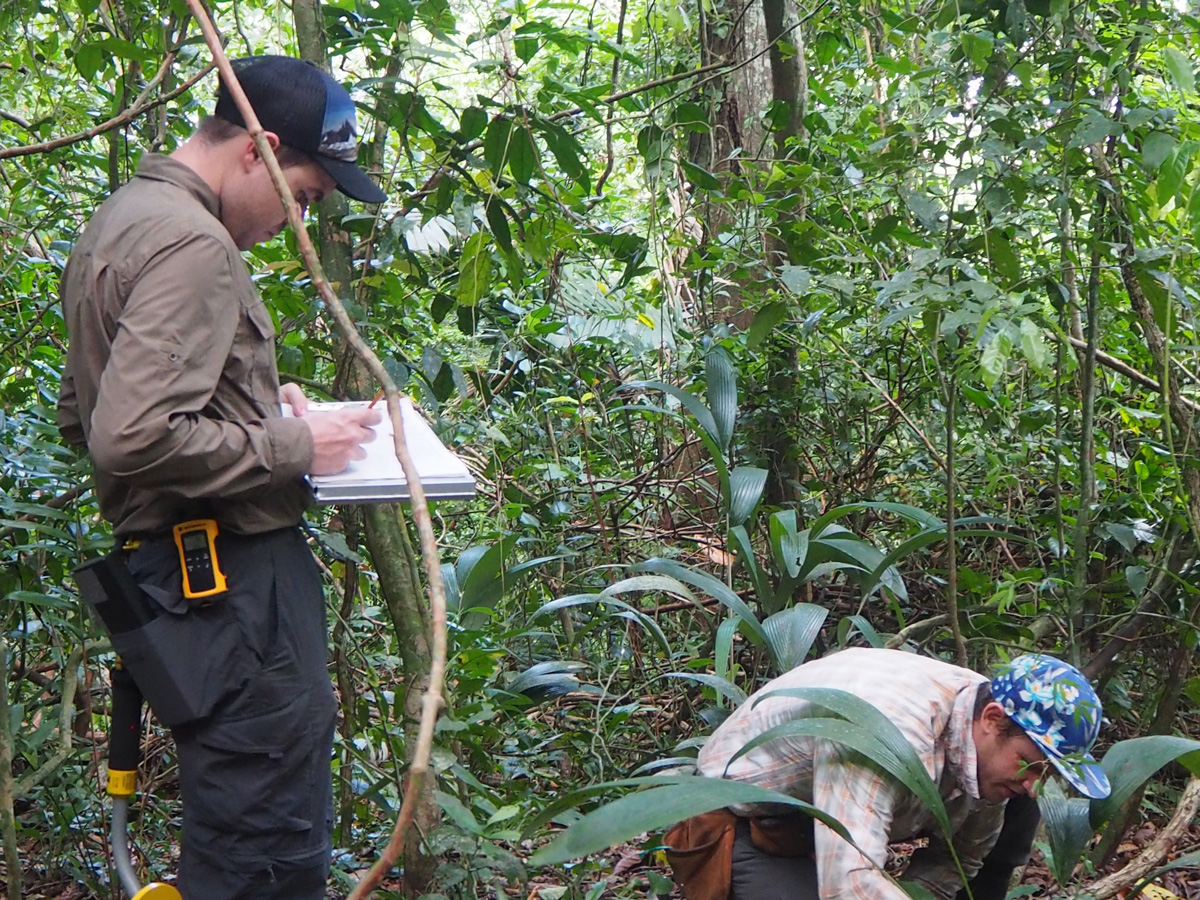
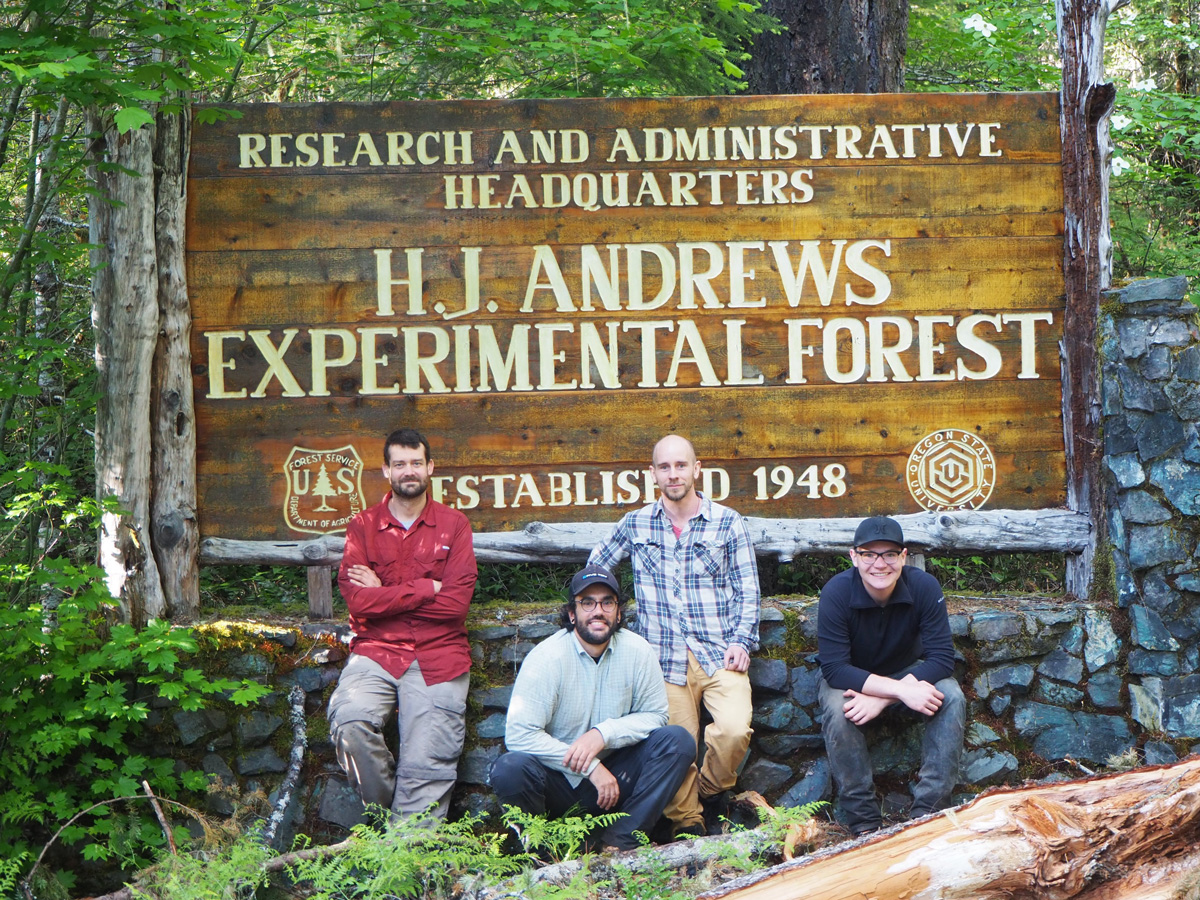
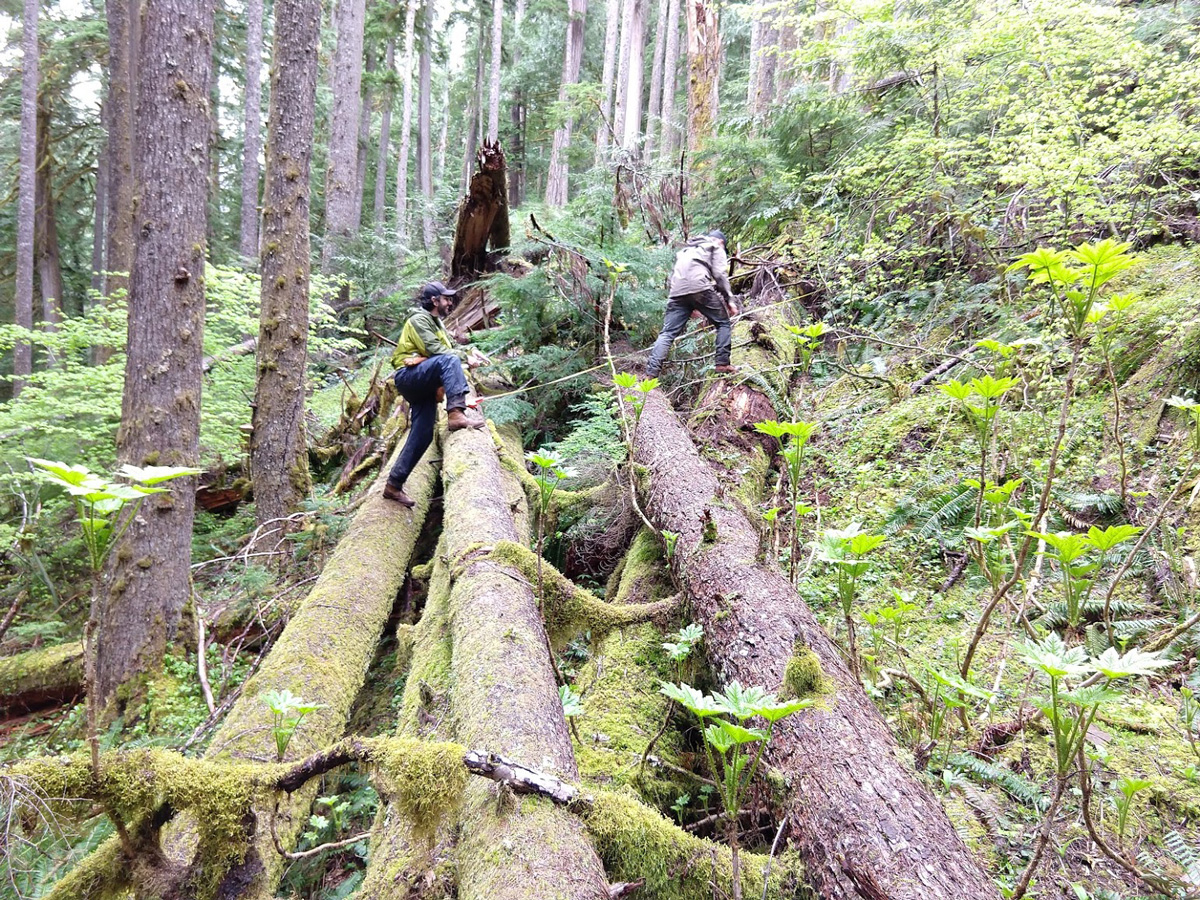
Key resources
Buzzard V, Michaletz ST, Deng Y, He Z, Ning D, Shen L, Tu Q, Van Nostrand J, Voordeckers JW, Wang J, Weiser MD, Kaspari M, Waide RB, Zhou J, Enquist BJ. 2019. Continental scale structuring of forest and soil diversity via functional traits. Nature Ecology & Evolution 3:1298-1308. Full text, Supplementary Information
Hogan J, McMahon S, Buzzard V, Michaletz ST, Enquist BJ, Thompson J, Swenson N, Zimmerman J. 2019. Drought and the interannual variability of stem growth in an aseasonal, everwet forest. Biotropica. Full text
Deng Y, Ning D, Qin Y, Xue K, Wu L, He Z, Yin H, Liang Y, Buzzard V, Michaletz ST, Zhou J. 2018. Spatial scaling of forest soil microbial communities across a temperature gradient. Environmental Microbiology 20:3504-3513. Full text
Zhou J, Deng Y, Shen L, Wen C, Qin Y, Xue K, Wu L, He Z, Voordeckers J, Buzzard V, Michaletz ST, Enquist BJ, Weiser MD, Kaspari M, Waide R, Yang Y, Brown JH. 2016. Temperature mediates continental-scale diversity of microbes in forest soils. Nature Communications 7:12083. Full text, Supplementary Material
Tu Q, Deng Y, Yan Q, He Z, Wu L, Buzzard V, Michaletz ST, Enquist BJ, Weiser MD, Kaspari M, Waide R, Brown JH, Shen L, Zhou J. 2016. Biogeographic patterns of soil diazotrophic communities across six forests in North America. Molecular Ecology 25:2937-2948. Full text
Plant Functional Traits Courses
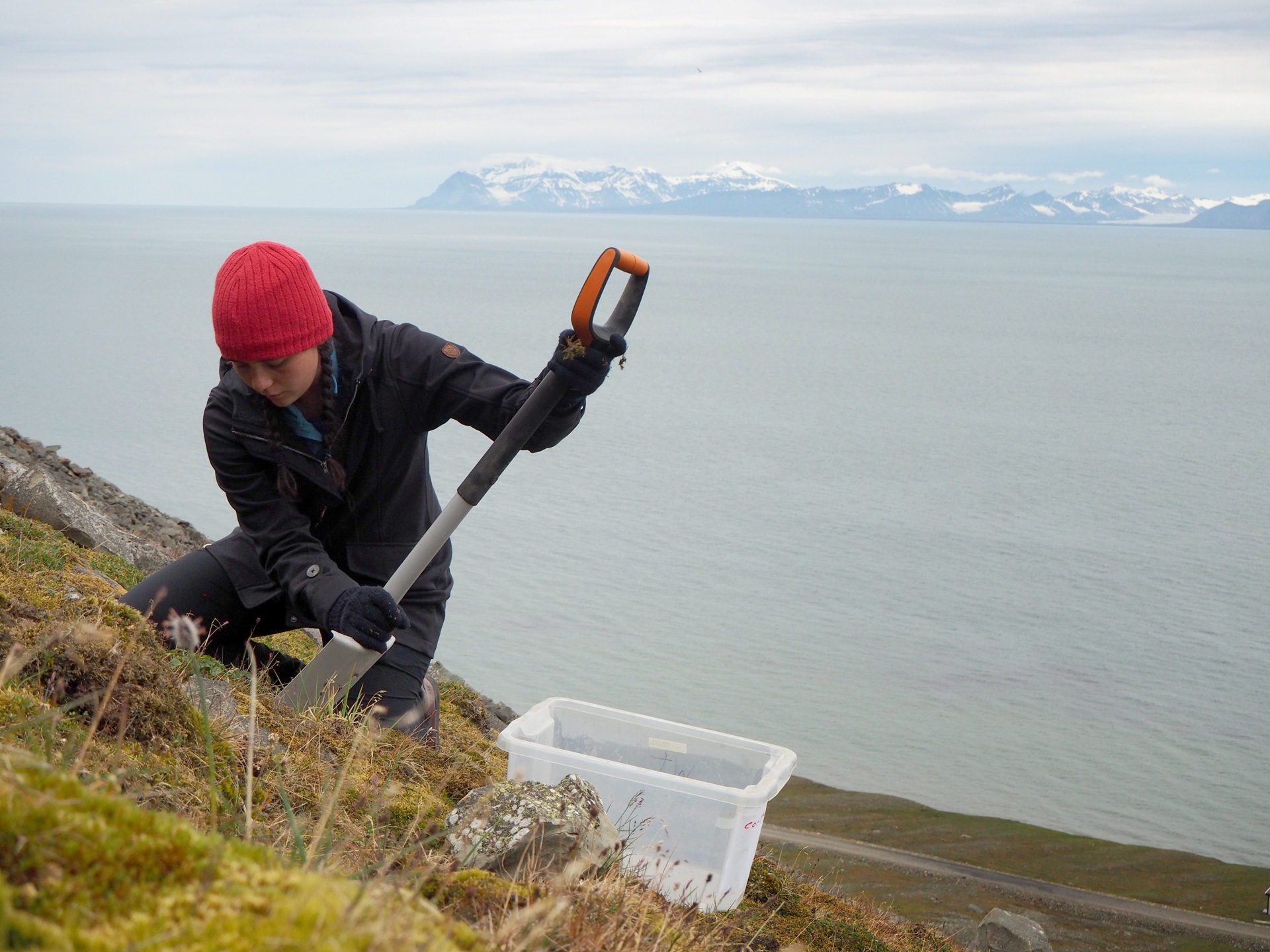
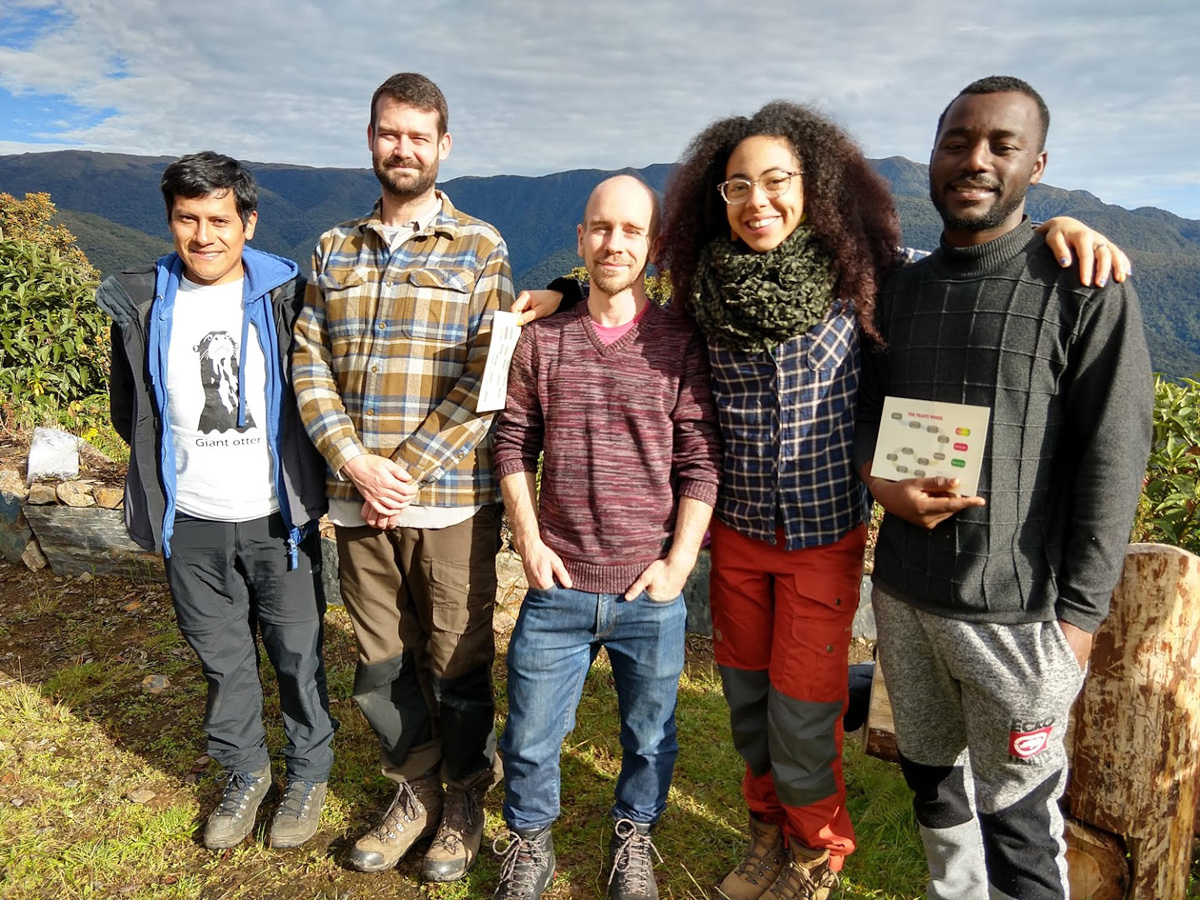
We have helped develop, organize, deliver and teach a series of international Plant Functional Traits Courses (PFTC) since the very beginning. The PFTC unite scientists of all career stages from around the world to collect, analyze, and publish functional ecology data. We have held five courses since 2015, in China, Peru, and Svalbard. The next two courses will be held in Norway and South Africa.
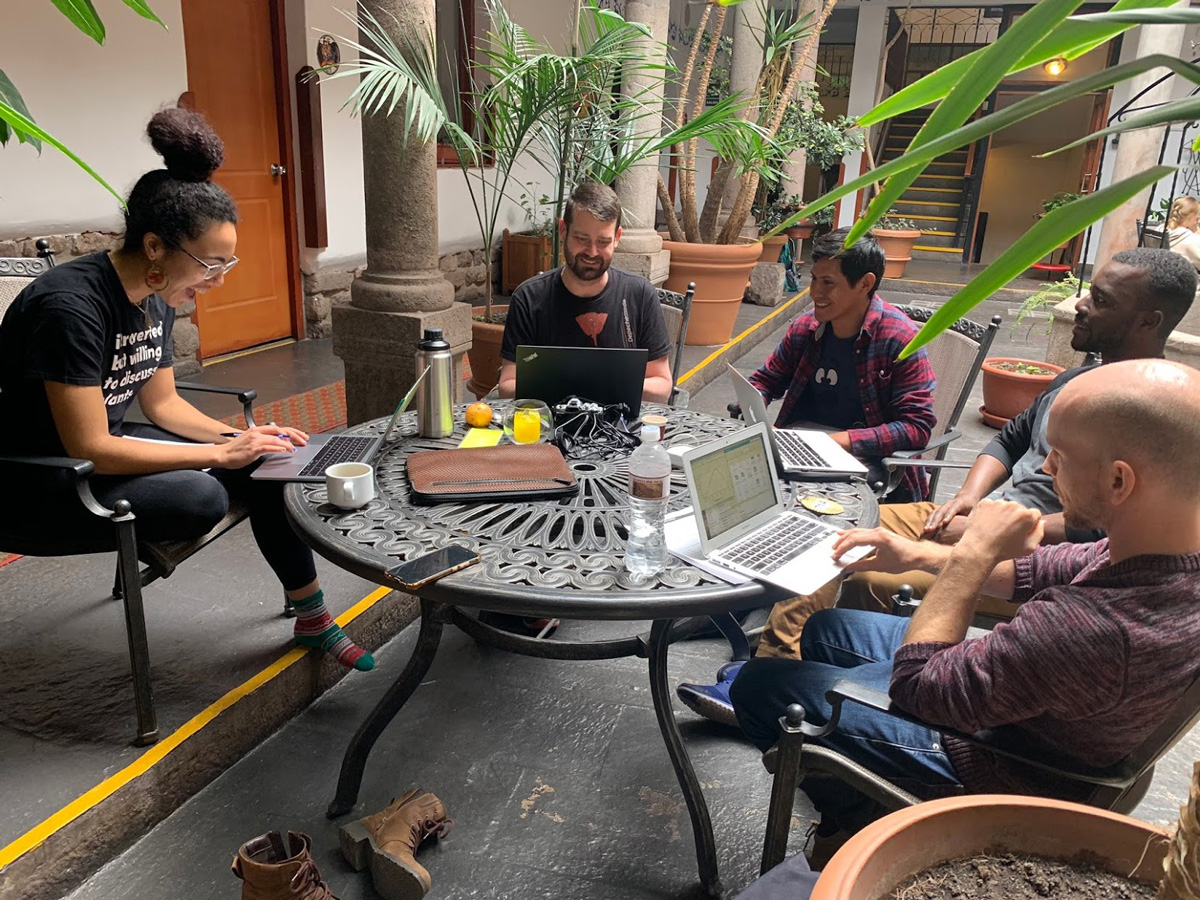
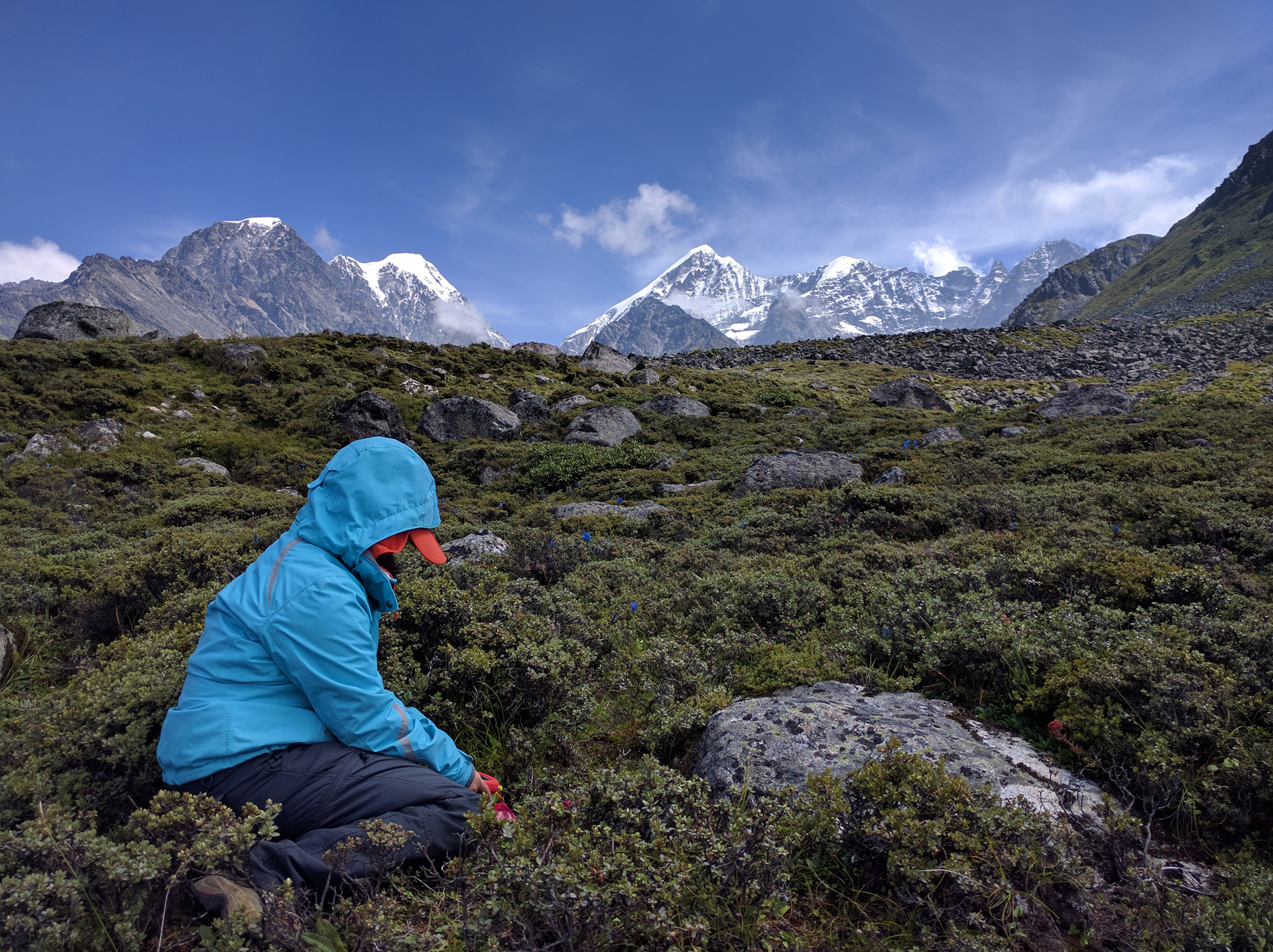
During the PFTCs, our lab has focused on the role of leaf traits and temperature in leaf metabolism. Specifically, we have collected data on leaf energy balance traits and photosynthesis temperature response from diverse taxa spanning large elevational climate gradients at each site. These data characterize the kinetics of leaf metabolism, and are being used to understand how climate effects on leaf metabolism “scale up” to control higher level processes such as plant growth and ecosystem production.
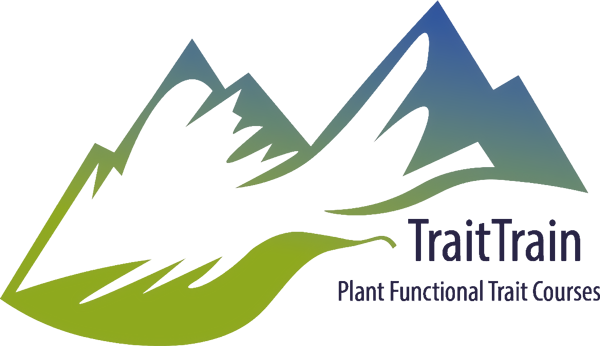
Key resources
Vandvik V, …, Michlaetz ST, Garen JC, …, and co-authors. 2024. Plant trait and vegetation data along a 1314 m elevation gradient with fire history in Puna grasslands, Perú. Scientific Data 11:225. Full text
Jónsdóttir IS, Halbritter AH, Henn JJ, Althuizen IHJ, Björnsdóttir K, Christiansen CT, Haugum SV, Maitner BS, Malhi Y, Michaletz ST, Roos RE, Klanderud K, Lee H, Enquist BJ, Vandvik V. 2022. Intraspecific trait variability is a key feature underlying high Arctic plant community resistance to climate warming. Ecological Monographs e1555. Full text
Thomson E, Spiegel M, Althuizen I, Bass P, Chen S, Chmurzynski A, Halbritter A, Henn J, Jónsdóttir I, Klanderud K, Li Y, Maitner B, Michaletz ST, Niittynen P, Roos R, Telford R, Enquist B, Vandvik V, Macias-Fauria M, Malhi Y. 2021. Multiscale mapping of plant functional groups and plant traits in the High Arctic using handheld spectroscopy, UAV imagery and Sentinel-2A data. Environmental Research Letters 16:055006. Full text
Vandvik V, …, Michaletz ST, and 29 co-authors. 2020. Plant traits and vegetation data from climate warming experiments along an 1000 m elevation gradient in Gongga Mountains, China. Scientific Data. Full text
Geange SR, von Oppen J, Strydom T, Boakye M, Gauthier T-LJ, Gya R, Halbritter AH, Jessup LH, Middleton SL, Navarro J, Pierfederici ME, Enquist BJ, Farfan-Rios W, Maitner BS, Michaletz ST, Telford RJ, Cotner S, Vandvik V. 2020. Next generation field courses: integrating Open Science and online learning. Ecology & Evolution 00:1-11. Full text
Henn JJ, Buzzard V, Enquist BJ, Halbritter AH, Klanderud K, Maitner BS, Michaletz ST, Pötsch C, Seltzer L, Telford RJ, Yang Y, Zhang L, Vandvik V. 2018. Intraspecific trait variation and phenotypic plasticity mediate alpine plant species response to climate change. Frontiers in Plant Science 9:1548. Full text
Drivers of terrestrial ecosystem processes
A central challenge facing science is understanding how the environment influences plant physiology across scales. While it’s been known for more than a century that physiological rates are influenced by temperature, predicting emergent outcomes at higher levels of organization is more complex. Indeed, understanding how climate influences metabolism and how this ‘scales up’ to higher levels is a realm of intense research.
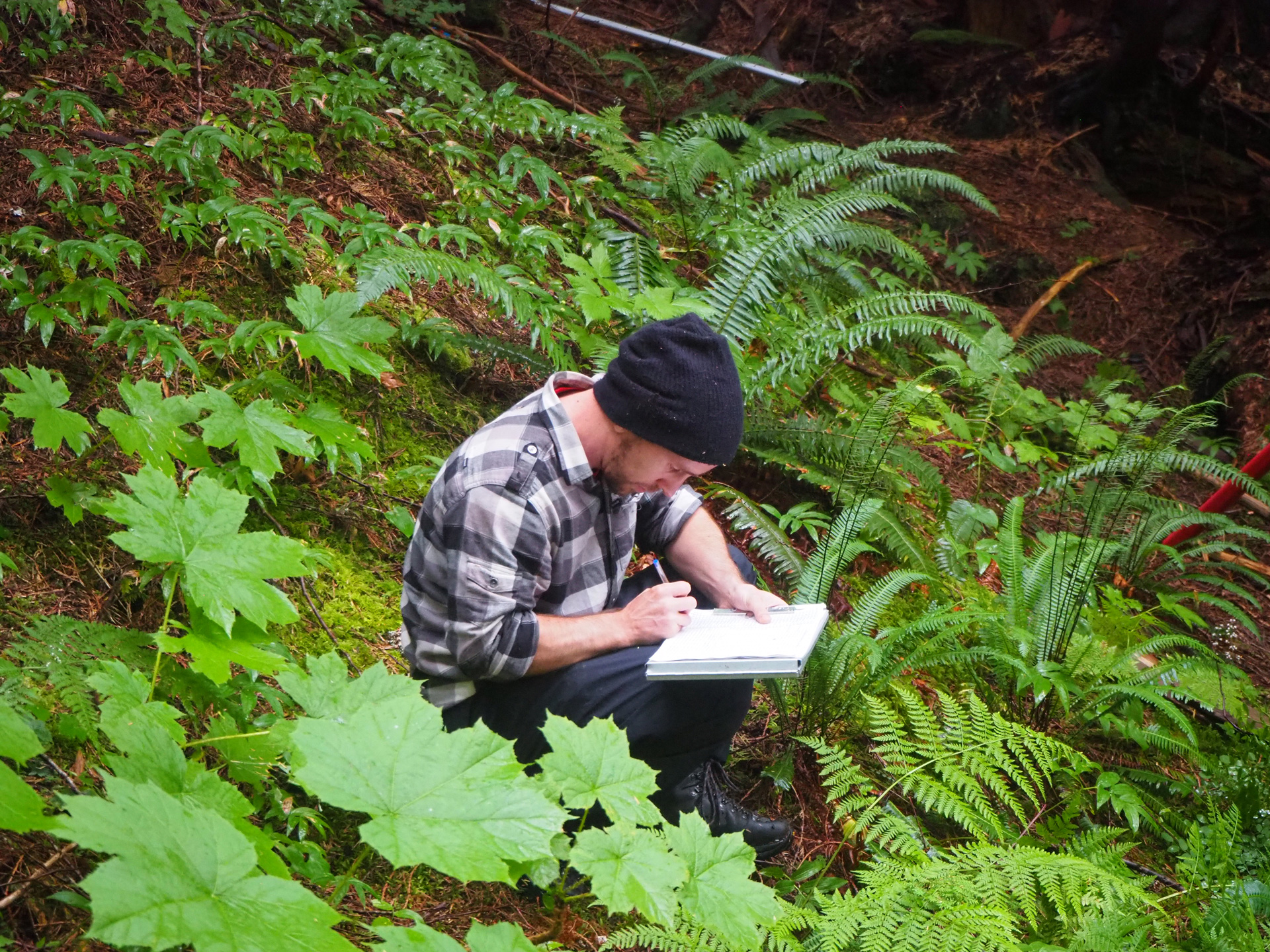
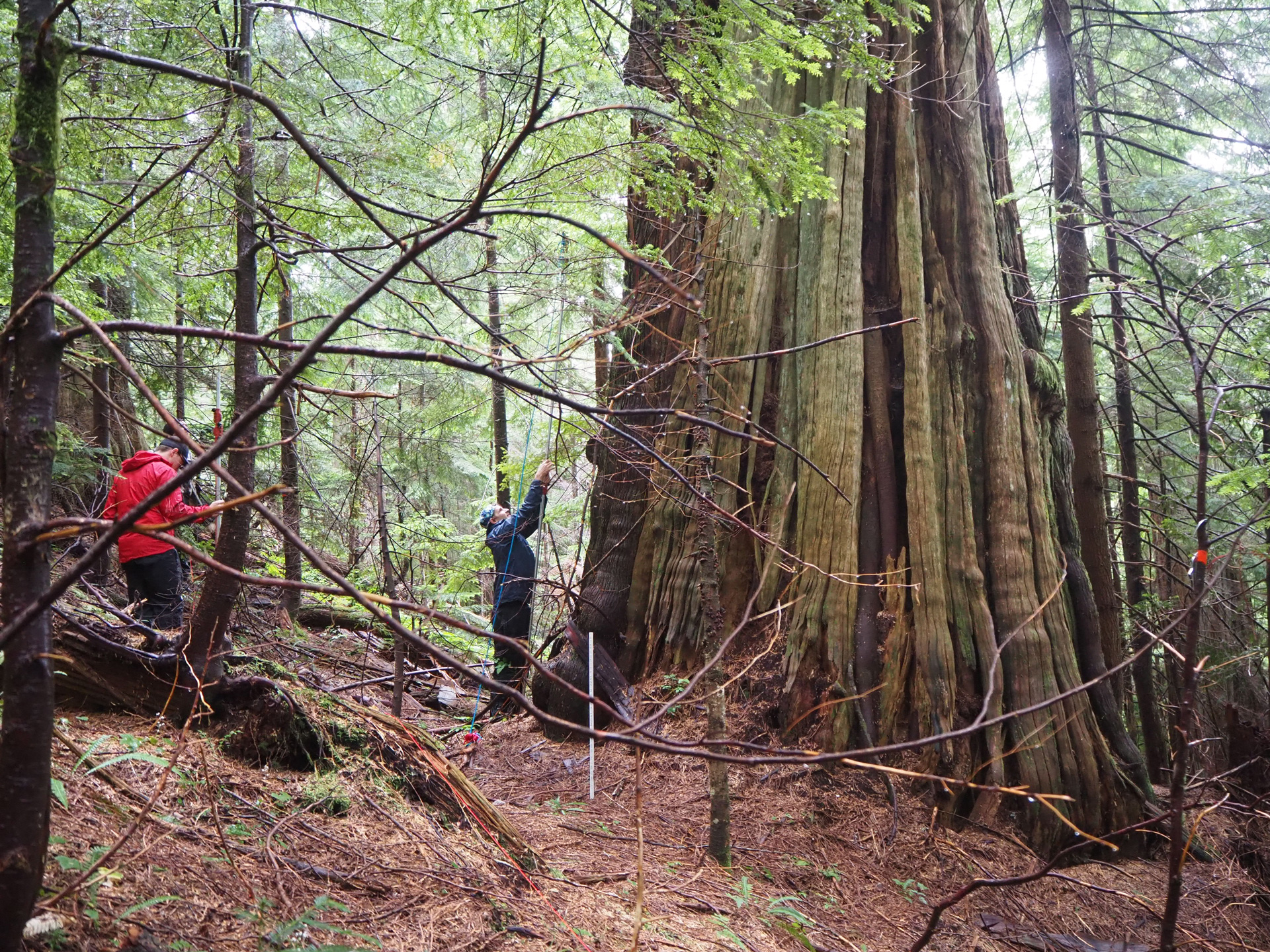
Temperature influences plant growth via kinetic effects on metabolism and has been proposed to obey a general Arrhenius relationship. However, this is complicated by adaptive responses of biochemistry that compensate for climate variation. Further, local adaptation of plant traits acts via energy budgets, ensuring that plant microclimates remain relatively close to photosynthetic optima. While we have known that plant microclimates are decoupled from macroclimate, recent work by us and others has documented a general homeostasis of plant microclimates.
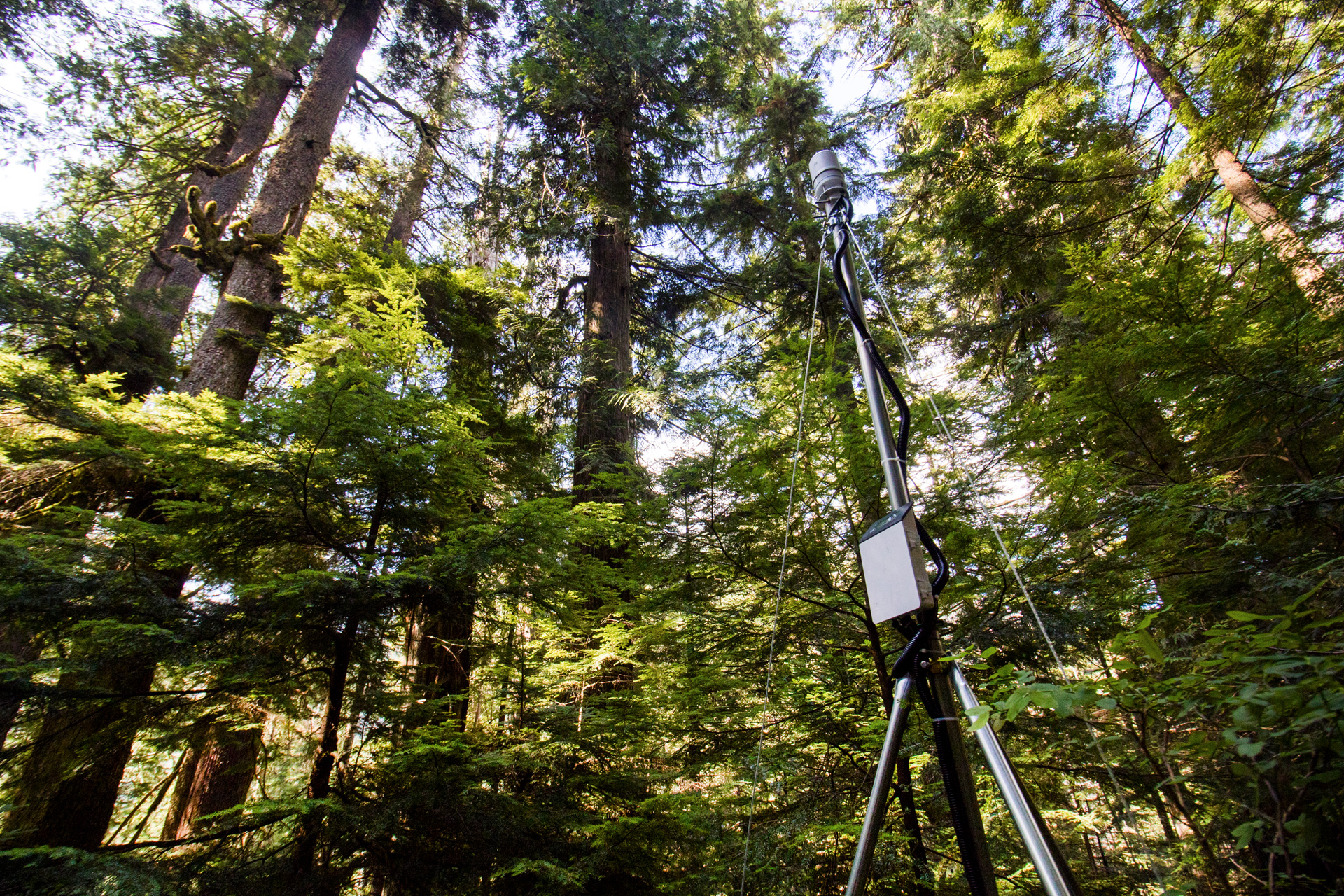
Key resources
Garen JC, Michaletz ST. In press. Acclimation unifies the scaling of carbon assimilation across climate gradients and levels of organization. Ecology Letters. Full text, Data, Code
Hu Z, Fernandez-Martinez M, He Q, Xu Z, Zhou G, Chen J, Nie M, Yu Q, Feng H, Huang Z Michaletz ST. 2024. Fungal composition associated with host tree identity mediates nutrient addition effects on wood microbial respiration. Ecology. Full text
Garen JC, Aparecido LMT, Blonder BW, Cavaleri MA, Slot M, Michaletz ST. 2023. Canopy-top measurements do not accurately quantify canopy-scale leaf thermoregulation. Proceedings of the National Academy of Sciences of the USA 120:e2301914120. Full text
Hu Z, Chen H, Gong XY, Shao J, Zhou G, Wang J, Yue C, Wang M, Xia J, Li Y, Zhou X, Michaletz ST. 2020. Traits mediate drought effects on wood carbon fluxes. Global Change Biology 26:3429-3442. Full text, Supplementary Information
Hu Z, Michaletz ST, Johnson DJ, McDowell NG, Huang Z, Zhou X, Xu C. 2018. Traits drive global wood decomposition rates more than climate. Global Change Biology 24:5259-5269. Full text, Appendix S1, Source Data S2, Appendix S3
Michaletz ST. 2018. Evaluating the kinetic basis of plant growth from organs to ecosystems. New Phytologist 219:37-44. Full text, Supporting Information, R code
Michaletz ST, Kerkhoff AJ, Enquist BJ. 2018. Drivers of terrestrial plant production across broad geographical gradients. Global Ecology and Biogeography 27:166-174. Full text, Supplementary Information, Source Data
McDowell NG, Michaletz ST, Bennett KE, Solander KC, Xu C, Maxwell RM, Middleton RS. 2018. Predicting chronic climate-driven disturbances and their mitigation. Trends in Ecology & Evolution 33:15-27. Full text, Supplement
Michaletz ST, Cheng D, Kerkhoff AJ, Enquist BJ. 2014. Convergence of terrestrial plant production across global climate gradients. Nature 512:39-43. Full text, Supplementary Information, Source Data, Corrigendum
Wildfire effects on plants
It is often thought that a wildfire will consume and kill all of the vegetation within its perimeter, but this is more an exception than a rule. Indeed, heterogeneity of fuels and microclimate leads to heterogeneity of fire behavior and effects, so that injured but surviving plants often remain after a wildfire. This has important emergent outcomes spanning levels of biological organization, from cellular photosynthesis and respiration to ecosystem production and evapotranspiration. However, despite more than half a century of research, the mechanisms by which fire injuries occur and interact are not well understood.
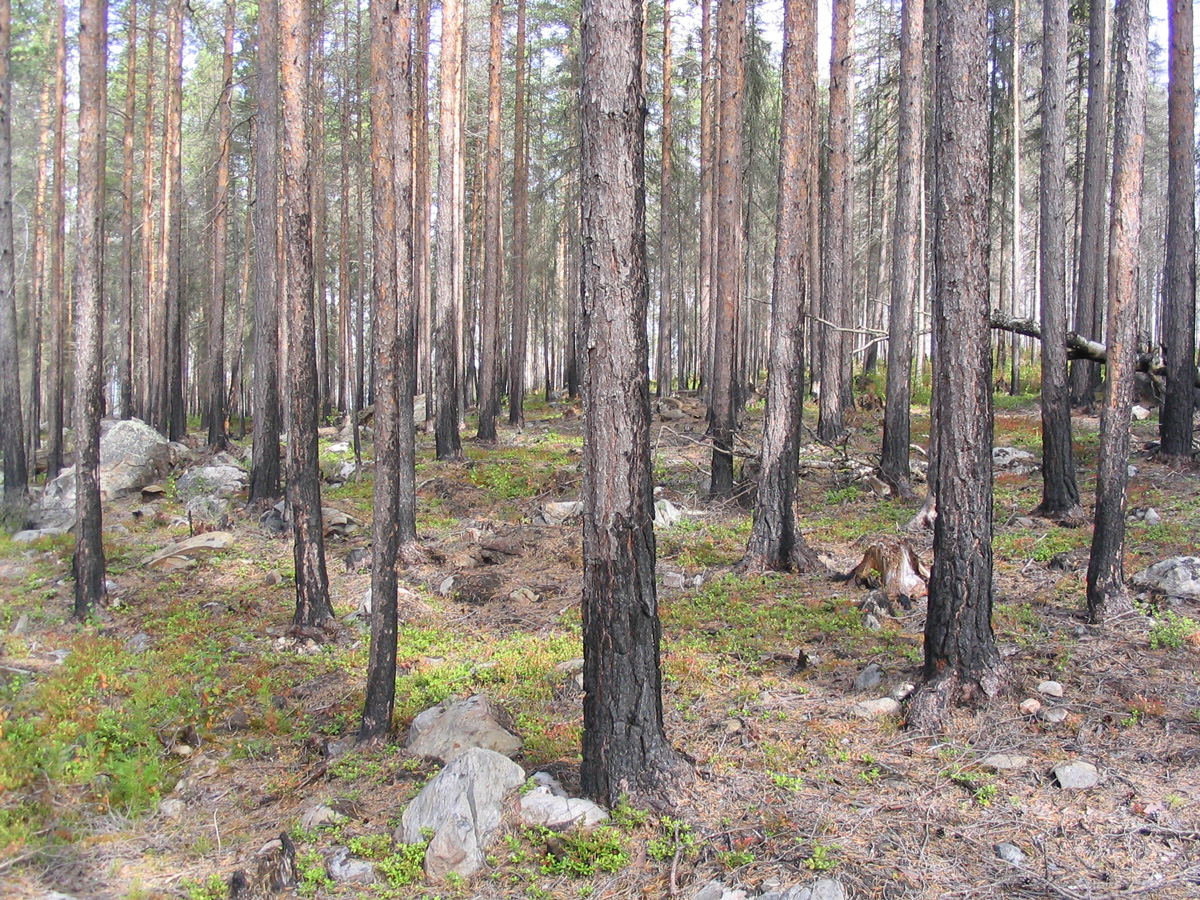
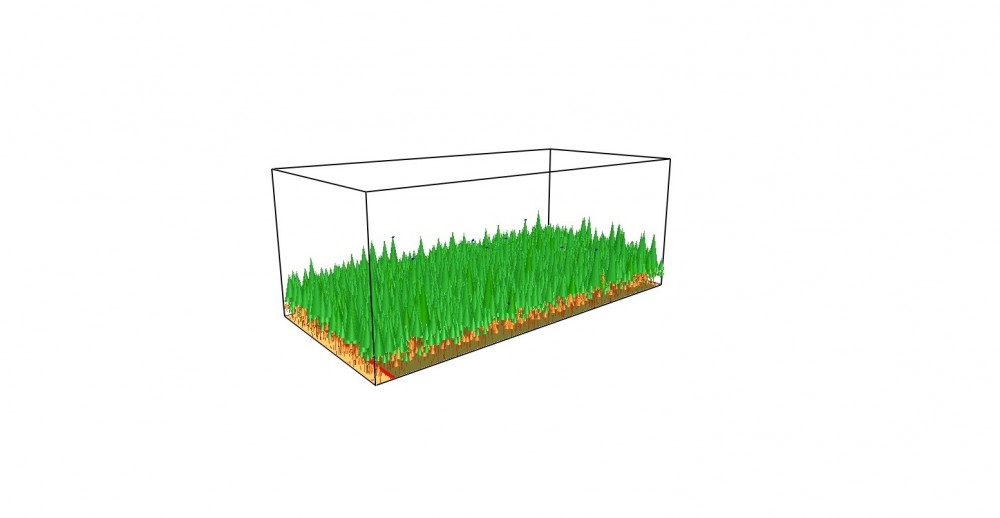
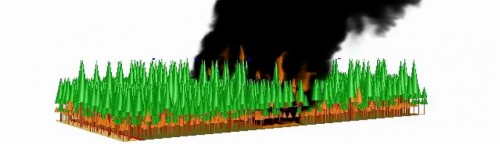
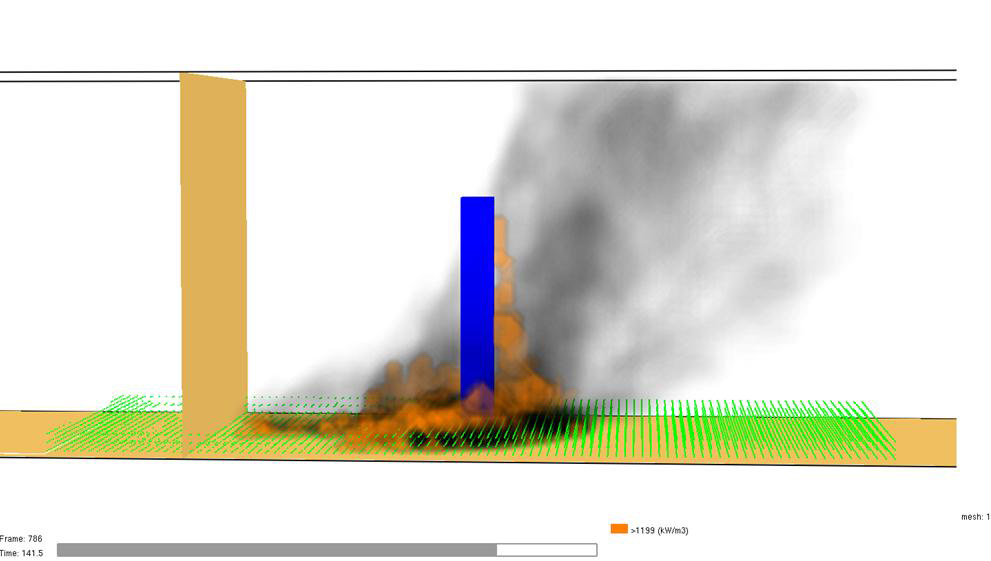
For more than two decades, we have been studying the effects of wildfires on plants. Our work focuses on the physical mechanisms linking wildfire behavior to plant physiology, and how fire effects “scale up” across levels of biological organization, from cells to ecosystems. We use a variety of approaches, including field and laboratory experiments, mathematical modelling, and highly parallelized High Performance Computing simulations.
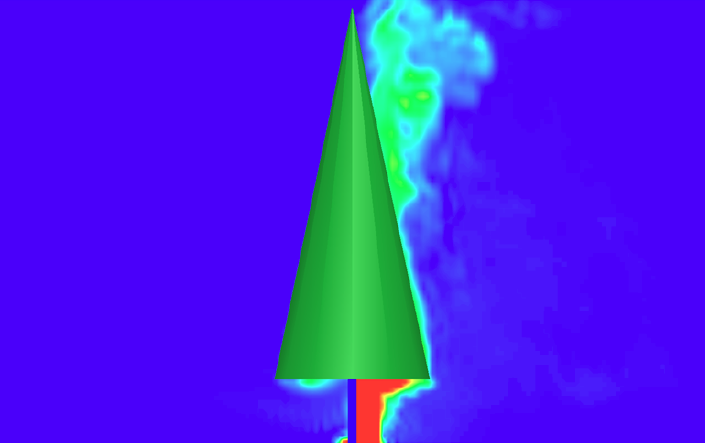
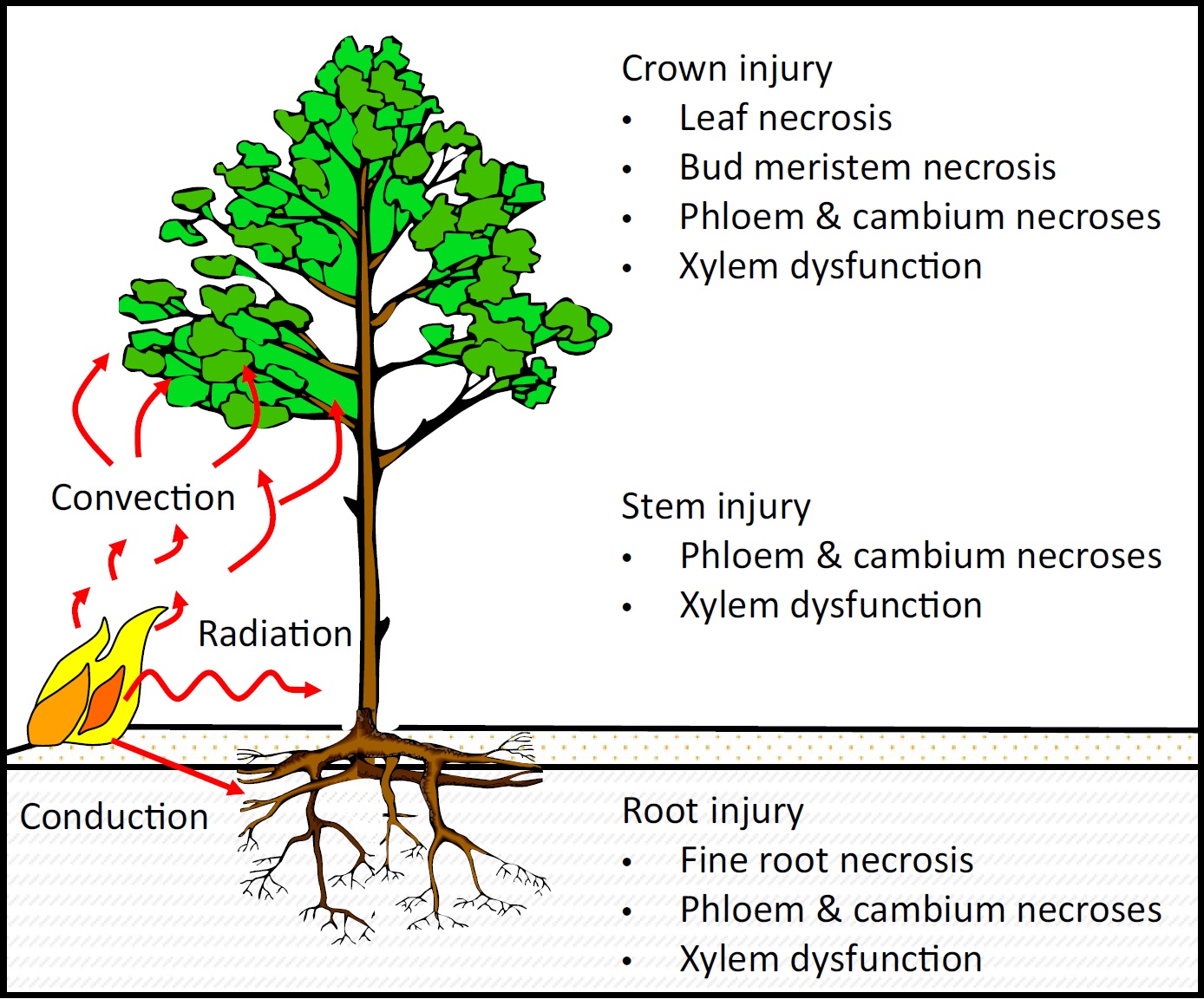
Key resources
Greene DF, Kane JM, Pounden E, Michaletz ST. 2024. Cone allometry and protection of seeds from fire are similar for serotinous and non-serotinous conifers. New Phytologist 242:93-106. Full text, Supporting Information, FDS input and output files
Bison NN, Partelli Feltrin R, Michaletz ST. 2022. Trait phenology and fire seasonality co-drive seasonal variation in fire effects on tree crowns. New Phytologist 234:1654-1663. Full text, Supporting Information, Fire data, Trait data, Convection data, Code
Kleynhans EJ, Atchley A, Michaletz ST. 2021. Modelling fire effects on plants: From organs to ecosystems. In Johnson EA, Miyanishi K (eds). Plant Disturbance Ecology: The Process and the Response (2nd ed). pp. 383-421. Full text
Bär A, Michaletz ST, Mayr S. Fire effects on tree physiology. 2019. New Phytologist 223:1728-1741. Full text
Michaletz ST. 2018. Xylem dysfunction in fires: Towards a hydraulic theory of plant responses to multiple disturbance stressors. New Phytologist 217:1931-1393. Full text